Have you ever wondered how a single parent plant can produce offspring with a mix of traits? Perhaps you’ve gazed at a garden overflowing with different flower colors, sizes, and petal shapes, marveling at the diversity of life. The answer lies in the fascinating world of genetics, where the intricate dance of genes determines the characteristics we inherit. In this article, we’ll delve into the intricacies of dihybrid crosses, exploring the fundamental concepts that govern these complex genetic patterns. We’ll unpack chapter 10’s practice problems, providing you with the answers and insights to become a true master of genetics.
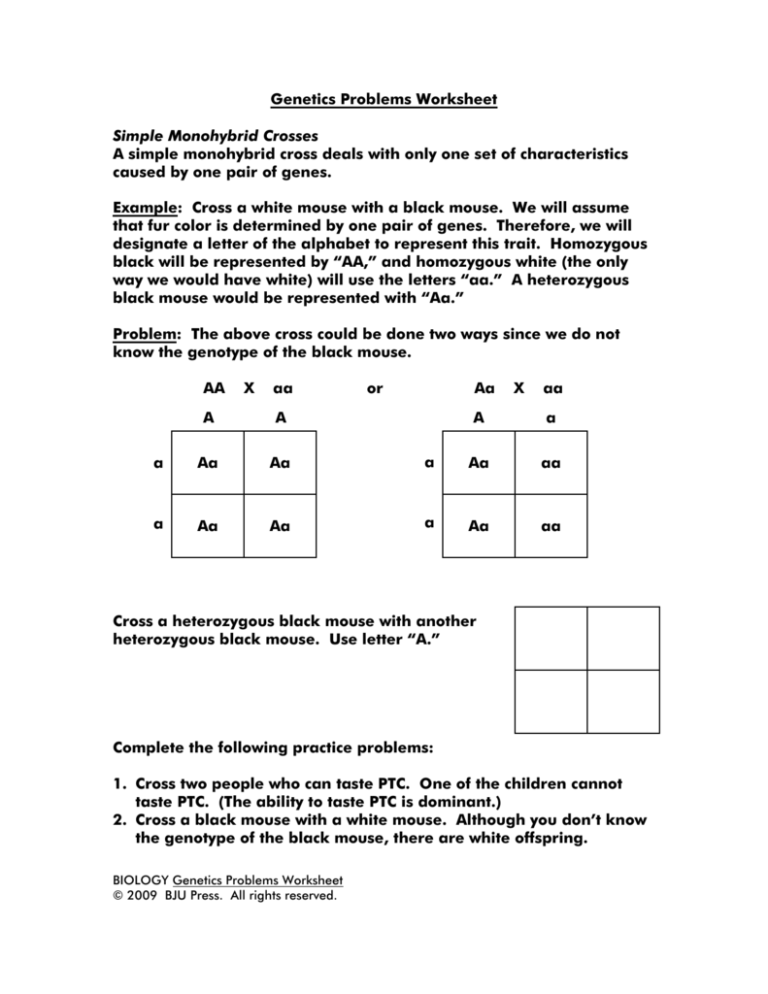
Image: printablezoneesther.z13.web.core.windows.net
Dihybrid crosses, involving the inheritance of two different traits, often leave students stumped. But, fear not! By understanding the basic principles of Mendelian genetics, you’ll unlock the secrets behind these intricate genetic combinations. Picture a garden with pea plants boasting both purple flowers and tall stems. Imagine crossing these two traits to discover the potential outcomes of their offspring. This is where the power of dihybrid crosses shines, allowing us to predict the probabilities of various combinations in the next generation. As we embark on this journey, remember that genetics is not just about memorizing rules; it’s about understanding the fundamental principles that govern life’s beautiful diversity.
Deciphering Dihybrid Crosses: A Journey into Genetic Inheritance
Before diving into the practice problems, let’s first understand the core concepts behind dihybrid crosses. We’ll start with the iconic work of Gregor Mendel, the father of genetics who laid the foundation for our understanding of inheritance. Mendel’s groundbreaking experiments with pea plants revealed the fundamental laws of heredity, which we still use today. His “Law of Independent Assortment” states that different genes (such as those controlling flower color and stem height) segregate independently during gamete formation, leading to the possibility of multiple combinations in the offspring.
Imagine each gene as a coin, with two sides representing different alleles – variations of a gene. In a dihybrid cross, we deal with two pairs of alleles, making the possibilities even more intriguing. For instance, consider the “R” gene controlling flower color, with “R” representing purple and “r” representing white. Similarly, “T” represents tall stem height while “t” represents short stem height.
The parental generation (P) is our starting point where we cross two homozygous individuals, one with both dominant traits (RRTT: purple flowers and tall stem) and the other with both recessive traits (rrtt: white flowers and short stem). Each parent will produce only one type of gamete – “RT” for the homozygous dominant parent and “rt” for the homozygous recessive parent. Their offspring, the F1 generation, will all inherit both dominant alleles, resulting in the genotype “RrTt” and phenotype of purple flowers and tall stems.
The real excitement begins in the F2 generation when we cross two individuals from the F1 generation. Here’s where the magic happens, as the gametes they produce can possess various combinations of alleles: “RT,” “Rt,” “rT,” and “rt.” This leads to a diverse mix of genotypes in the F2 generation, resulting in a beautiful display of phenotypic variation.
Unveiling the Answer Key: Deconstructing Chapter 10’s Practice Problems
Now that we’ve delved into the theoretical framework, let’s tackle those practice problems from Chapter 10 head-on. Each scenario will present a unique genetic puzzle, requiring us to apply the principles of dihybrid crosses and Punnett squares to predict the probabilities of different phenotypes in the offspring. These practice problems are like treasure hunts, leading us to a deeper understanding of the intricate interplay of genes.
Problem 1: A homozygous pea plant with purple flowers and tall stems (RRTT) is crossed with a homozygous pea plant with white flowers and short stems (rrtt). What are the genotypes and phenotypes of the F1 generation?
Answer: Since both parents are homozygous, they can only produce one type of gamete: RT and rt, respectively. Their F1 offspring will all be heterozygous for both traits – RrTt. These offspring will all have purple flowers and tall stems, due to the dominant alleles.
Problem 2: Two F1 pea plants from Problem 1 are crossed (RrTt x RrTt). Determine the genotypic and phenotypic ratios of the F2 generation.
Answer: This is where the Punnett square comes in handy. We create a 4×4 grid, representing all possible combinations of gametes from each parent (RT, Rt, rT, rt). Filling in the grid, we can see that the F2 generation has 9 distinct genotypes:
- RRTT: 1/16 chance (purple flowers, tall stem)
- RRTt: 2/16 chance (purple flowers, tall stem)
- RRtt: 1/16 chance (purple flowers, short stem)
- RrTT: 2/16 chance (purple flowers, tall stem)
- RrTt: 4/16 chance (purple flowers, tall stem)
- Rrtt: 2/16 chance (purple flowers, short stem)
- rrTT: 1/16 chance (white flowers, tall stem)
- rrTt: 2/16 chance (white flowers, tall stem)
- rrtt: 1/16 chance (white flowers, short stem)
Therefore, the phenotypic ratio is 9:3:3:1, meaning 9 out of 16 offspring will have purple flowers and tall stems, 3 out of 16 will have purple flowers and short stems, 3 out of 16 will have white flowers and tall stems, and 1 out of 16 will have white flowers and short stems.
Problem 3: A fruit fly with long wings and white eyes (WwEe) is crossed with a fruit fly with short wings and red eyes (wwEE). What proportion of the offspring are expected to have both long wings and red eyes?
Answer: We need to identify the genotypes that produce the desired phenotype: long wings (W_) and red eyes (EE). Setting up our Punnett square, we see that the offspring genotypes with both traits are:
- WwEE
- WwEe
Adding those probabilities together, we find that 5/8 or 62.5% of the offspring are expected to have both long wings and red eyes.
These examples illustrate the power of dihybrid crosses in predicting offspring characteristics. By understanding the principles of independent assortment and using the Punnett square to visualize the possible gamete combinations, we can confidently decipher any genetic puzzle.
Beyond the Textbook: Applying Dihybrid Crosses in Real Life
These principles are not merely theoretical exercises; they have real-world implications. From agriculture to medicine, understanding dihybrid crosses can revolutionize our lives.
Breeders can use this knowledge to develop crops with desirable traits like higher yield, disease resistance, and pest tolerance. The principles of dihybrid crosses can also be used to determine the chances of inheriting genetic diseases. By tracking the inheritance of specific traits within families, healthcare professionals can advise individuals about potential risks or recommend genetic testing to gain a more comprehensive understanding of their genetic makeup.
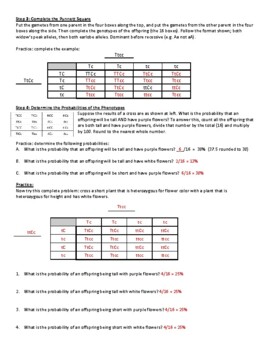
Image: learningschoolralf.z13.web.core.windows.net
Chapter 10 Dihybrid Practice Problems Answer Key
Embracing the Mystery: Exploring the Future of Genetics
Genetics is a dynamic field constantly evolving with exciting discoveries. Future advancements in genetic engineering offer the promise of treating genetic diseases and enhancing human capabilities. By delving into dihybrid crosses and mastering the concepts within Chapter 10, we unlock the potential to make a real difference in the world, contributing to innovations that will shape the future of healthcare, agriculture, and beyond.
As you delve deeper into the world of genetics, remember that the journey is never truly over. It’s about embracing the mystery, asking questions, and seeking answers. Just like with each dihybrid cross, we uncover new insights that reveal the immense complexity and beauty of life. So, go forth, armed with your knowledge of dihybrid crosses, and explore the fascinating world of genetics – a world where every cell tells a story of inheritance, resilience, and life’s breathtaking complexity.